In a recent experiment, the scientific community received a resounding affirmation of Albert Einstein’s profound insight. This experiment dismantled the notion of antigravity and debunked hopes of harnessing antimatter for levitation or constructing perpetual motion machines or warp drives. Einstein’s Theory Confirmed: Antigravity Challenged. The implications for sci-fi enthusiasts and “Star Trek” fans may be disheartening, but the results carry significant implications for our understanding of the fundamental forces at play in the universe.
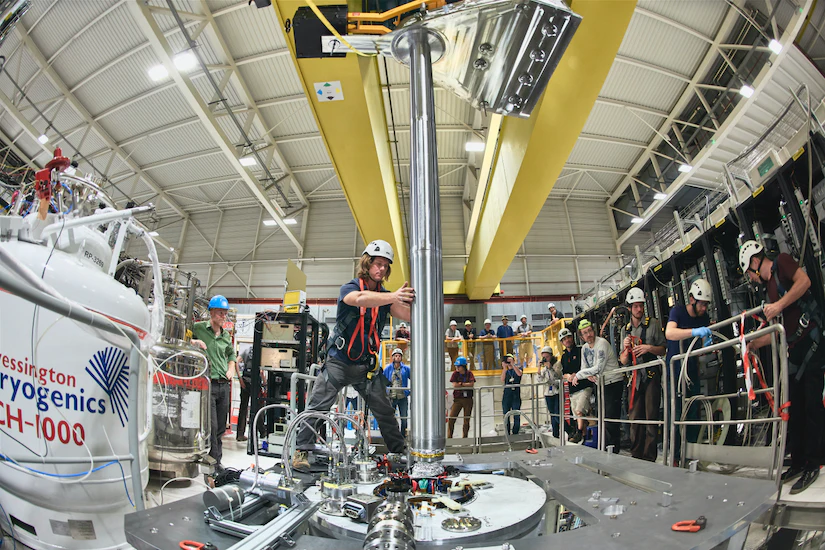
The reality of antimatter
Antimatter is an intriguing and tangible aspect of the universe. Comprising particles that mimic regular matter in most respects, antimatter diverges due to the reversal of their electrical charges. For instance, an anti-proton shares similarities with a proton, but it bears a negative charge. Conversely, an anti-electron, or positron, closely resembles an electron but possesses a positive charge. When antimatter encounters matter, their interaction results in a spectacular release of energy, entirely converting their combined mass into a formidable display of energy.
Challenging Antigravity Theories
In a nutshell, the experiment involved dropping antihydrogen atoms through a tube, and they behaved exactly as Einstein had predicted: they were subject to gravity. Until this moment, a lingering possibility existed that antimatter might not respond to Earth’s gravity or that matter and antimatter experienced a sort of antigravity, pushing each other apart. To make antimatter, you need to combine positrons and antiprotons. Antiprotons are made when high-energy particles collide and are then slowed down at CERN’s antiproton decelerator. Positrons are generated through the radioactive decay of specific chemical elements like potassium. Working with antimatter is a challenge, as it vanishes when in contact with ordinary matter. To address this, the researchers employed an eight-pole magnet to control the movement of anti-hydrogen atoms, keeping them away from the walls of their containment. Slower anti-atoms were trapped inside the magnetic field, while faster ones could still escape.
Understanding the Experiment
Upon turning the tube of captured antimatter vertically, the researchers observed that atoms moving downward along the magnetic field lines accelerated due to the influence of gravity, while those moving upward slowed down, also under the pull of gravity. The researchers couldn’t directly observe the antimatter’s behavior, but their instruments detected the tiny energy bursts when an anti-hydrogen atom, pulled downward by gravity, gained enough speed to escape the magnetic field at the container’s bottom. This process resulted in the annihilation of antimatter and an ordinary matter atom.
The Implications of the Experiment
Approximately 80 percent of the anti-hydrogen atoms succumbed to Earth’s gravity, falling downward. The remaining 20 percent continued bouncing upward at sufficient speed to defy gravity. This outcome is consistent with what one might anticipate for a group of regular hydrogen atoms in a magnetic field, demonstrating that gravity affects both matter and antimatter in the same way. In essence, the experiment validated that matter and antimatter are attracted to each other, despite their unique properties.
While this outcome may seem predictable to physicists, it underscores the necessity of experimentation to explore potentially new physics. In the words of Jonathan Wurtele, a coauthor of the study from the University of California at Berkeley, “You don’t want to be the kind of stupid person who doesn’t do an experiment that explores possibly new physics because you thought you knew the answer, and then it ends up being something different.”
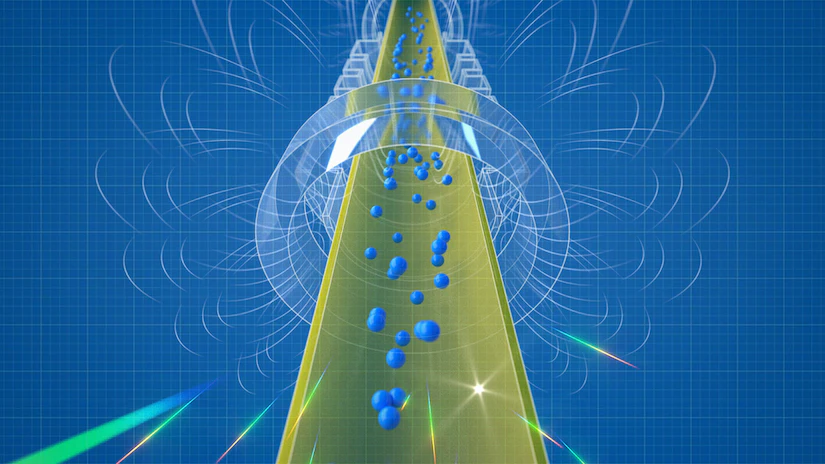
The Mystery of Missing Antimatter
The experiment’s results not only confirm Einstein’s predictions but also raise intriguing questions about the scarcity of antimatter in the observable universe. Einstein’s Theory Confirmed: Antigravity Challenged. According to general relativity, antimatter and matter should exist in equal quantities, yet antimatter is conspicuously absent, at least within our observable universe. This enigma prompts a fundamental question: where did all the antimatter go?
One hypothesis suggests that shortly after the Big Bang, matter and antimatter repelled each other significantly, effectively separating from each other, and leaving only isolated traces of scattered particles. However, Anderson and her colleagues’ experiment contradicts this idea, leaving scientists with a perplexing puzzle to unravel.
The road ahead
The experiment’s success paves the way for further investigations. By varying the strength of the magnetic field, researchers can precisely measure the acceleration of antimatter under gravity’s influence, ensuring that antimatter behaves comparably to regular matter in this respect. Currently, Anderson and her team are focused on exploring how antimatter interacts with radiation. If Einstein’s predictions hold, anti-hydrogen should absorb, emit, and reflect the same spectrum of light as regular hydrogen. Therefore, the next phase of their research involves subjecting antimatter to lasers and microwaves to scrutinize these interactions further.
This experiment reinforces Einstein’s enduring insights into the workings of the universe and challenges longstanding theories of antigravity. While it may not lead us to levitating cities or warp drives, it propels us toward a deeper understanding of the universe’s fundamental forces and the enigmatic nature of antimatter.
Remember, early detection is your strongest ally in the battle against breast cancer. Here’s to safeguarding your well-being and knowledge.
Read our previous articles: https://findcare.nuvancehealth.org/provider/Lisa+D.+Curcio/1746345
https://scitechupdate.com/index.php/zinc-should-get-from-food-not-supplements/
https://scitechupdate.com/index.php/three-nobel-prizes-try-to-cover-all-of-science/
https://scitechupdate.com/index.php/the-most-populated-cities-in-the-world/
https://scitechupdate.com/index.php/aromas-and-odors-decoding-the-insect-brains-interpretation/
https://scitechupdate.com/index.php/pig-kidney-xenotransplantation-is-thriving-in-human-body/
https://scitechupdate.com/index.php/huaweis-new-smartphone-challenger-to-apple/
https://scitechupdate.com/index.php/israel-advances-cancer-treatment-with-genomic-profiling/https://scitechupdate.com/index.php/stomach-cancer-causes-signs-and-treatment/
https://scitechupdate.com/index.php/james-webb-telescope-captures-newborn-sun-like-star/
https://scitechupdate.com/index.php/oxygen-28-unstable-magic-isotope-that-defies-expectations/